Exploring the Influence of Plate Tectonics on Biogeographic Regions, a scientific theory about the Earth’s crust, plays a vital role in shaping the world’s biogeographic regions. These regions are areas with distinct collections of plants and animals. By understanding how the Earth’s plates move and interact, we can uncover the secrets behind why certain species live where they do. Let’s delve into this fascinating connection.
Table of Contents
ToggleWhat are Biogeographic Regions
Biogeographic regions refer to large areas of the Earth’s surface with distinct collections of plants, animals, and other organisms that have evolved and adapted to the local environmental conditions over long period of time. These regions are characterized by specific patterns of biodiversity, influenced by factors such as climate, geography, and historical events. Biogeographic regions help scientists understand the distribution of life on Earth and the processes that shape it.
Plate tectonics is super important because it helps explain how the Earth’s surface changes over time. It’s like a giant puzzle where the pieces are Earth’s outer shell, or “plates,” and they’re always moving. This movement causes earthquakes, volcanic eruptions, and forms mountains and oceans. Understanding plate tectonics helps us predict natural disasters and learn more about Earth’s history.
Plate Tectonics Theory
Plate tectonics theory is the scientific theory that describes the movement and interaction of large fragments of the Earth’s lithosphere, known as tectonic plates. These plates, which vary in size and shape, float on the semi-fluid asthenosphere beneath them. The theory explains how these plates move over time, causing various geological phenomena such as earthquakes, volcanic eruptions, mountain formation, and the creation of ocean basins.
Types of plate tectonic boundaries:
There are three main types of plate tectonic boundaries:
- Divergent boundaries
- Convergent boundaries
- Transform boundaries
- Divergent boundaries: Divergent boundaries are areas where two tectonic plates are moving away from each other. This movement creates gaps in the Earth’s crust, allowing magma from the mantle to rise up and form new crust. As a result, divergent boundaries are often associated with the formation of mid-ocean ridges on the ocean floor and rift valleys on continents.
- Convergent boundaries: Convergent boundaries are places where two tectonic plates move toward each other, causing them to collide. This collision can lead to the formation of mountain ranges, earthquakes, and volcanic activity.
- Transform boundaries: Transform boundaries are areas where tectonic plates slide past each other horizontally. This movement can cause earthquakes and the formation of features like faults. An example of a transform boundary is the San Andreas Fault in California, where the Pacific Plate and the North American Plate slide past each other.
Connection between Plate Tectonics and Biogeographic Regions
Plate tectonics play a fundamental role in shaping biogeographic regions, which are areas characterized by distinct patterns of biodiversity and species distribution. The connection between plate tectonics and biogeography can be understood through several key mechanisms:
1. Continental Drift and Landmass Movement: Plate tectonics drive the movement of continents over geological time scales through processes such as seafloor spreading, subduction, and continental drift. As continents drift apart or come together, they create barriers to species dispersal and facilitate the formation of new habitats. This movement has led to the isolation of different biogeographic regions, allowing for the evolution of distinct flora and fauna.
2. Formation of Land Bridges and Corridors: Plate tectonics can create land bridges or corridors between previously isolated landmasses, enabling the exchange of species between biogeographic regions. For example, the formation and subsequent disappearance of land bridges, such as the Isthmus of Panama connecting North and South America, have had significant effects on the movement of species between the Americas and influenced the composition of their biotas.
3. Mountain Building and Habitat Diversification: Plate tectonics contribute to the formation of mountain ranges through processes like continental collision and uplift. Mountains act as barriers to species dispersal, leading to the formation of isolated habitats and promoting speciation. They also create a variety of microclimates and ecological niches, fostering high levels of biodiversity within relatively small geographic areas.
4. Ocean Basin Formation and Marine Biogeography: Plate tectonics shape the configuration of ocean basins and continental shelves, influencing ocean circulation patterns, sea level changes, and the distribution of marine habitats. These factors play a crucial role in determining the distribution of marine species and the formation of marine biogeographic regions, such as the delineation of tropical, temperate, and polar zones.
5. Volcanism and Island Biogeography: Plate tectonics are associated with volcanic activity, which can lead to the formation of islands and archipelagos. Islands provide unique opportunities for studying biogeography due to their isolated nature and limited species pools. The principles of island biogeography, developed by MacArthur and Wilson, emphasize the roles of colonization, extinction, and speciation in shaping species diversity on islands, which are influenced by factors such as island size, distance from the mainland, and habitat diversity.
Historical Context
Using the two important stages in the history of Earth’s continental configuration, reflecting the dynamic nature of plate tectonics and the ongoing movement of the Earth’s lithosphere over geological time scales: Pangea and Gondwana
- Pangea: Pangea was a supercontinent that existed during the late Paleozoic and early Mesozoic eras, about 335 to 175 million years ago. It was a vast landmass that included most of the Earth’s continental plates fused together. The concept of Pangea was proposed by Alfred Wegener in the early 20th century as part of his theory of continental drift. According to this theory, Pangea eventually broke apart due to the movement of tectonic plates, resulting in the continents we see today.
- Gondwana: Gondwana was a southern supercontinent that formed after the breakup of Rodinia, an earlier supercontinent, around 600 million years ago. It included what are now the continents of South America, Africa, Antarctica, Australia, the Indian subcontinent, and the Arabian Peninsula. Gondwana began to break apart during the Mesozoic era, leading to the formation of the modern continents in the Southern Hemisphere. The breakup of Gondwana played a crucial role in shaping the geological and biological history of the Earth, including the dispersal of species and the formation of new ocean basins.
Divergence and convergence process
Divergence and convergence are two fundamental processes in plate tectonics, which describe the movement of tectonic plates relative to each other:
- Divergence: Divergence occurs when tectonic plates move away from each other. This movement typically happens along mid-ocean ridges, where magma rises from the mantle to create new crust. As the magma cools and solidifies, it forms new oceanic crust, causing the plates on either side to move apart. Divergent boundaries are characterized by features such as rift valleys and volcanic activity. One of the most well-known examples of divergence is the Mid-Atlantic Ridge, where the Eurasian Plate and the North American Plate are moving apart, creating new oceanic crust between them.
- Convergence: Convergence occurs when tectonic plates move towards each other. This movement results in the collision and interaction of the plates, leading to various geological features and phenomena. There are three main types of convergence:
- Oceanic-Continental Convergence: This type of convergence happens when an oceanic plate collides with a continental plate. The denser oceanic plate is forced beneath the less dense continental plate in a process called subduction. This can lead to the formation of deep ocean trenches, volcanic arcs, and mountain ranges. An example of oceanic-continental convergence is the Andes mountain range in South America, where the Nazca Plate is subducting beneath the South American Plate.
- Oceanic-Oceanic Convergence: This occurs when two oceanic plates converge. One plate typically subducts beneath the other, forming deep ocean trenches and volcanic island arcs. The collision can also result in the formation of underwater mountain ranges. An example is the collision between the Pacific Plate and the Philippine Sea Plate, creating the Mariana Trench and the Mariana Islands.
- Continental-Continental Convergence: When two continental plates converge, neither plate subducts due to their low density. Instead, the collision can cause the crust to fold and buckle, leading to the formation of large mountain ranges. The Himalayas, formed by the collision of the Indian Plate and the Eurasian Plate, are a prominent example of continental-continental convergence.
Formation of Habitats and Barriers
The formation of habitats and barriers is intimately linked to geological processes, including the movement of tectonic plates, the shaping of landforms by erosion and weathering, and the distribution of water bodies. Here’s how these processes contribute to the formation of habitats and barriers:
- Tectonic Plate Movement: The movement of tectonic plates can lead to the formation of mountains, valleys, and other landforms, creating diverse habitats and barriers for species. For example, the collision of continental plates can create towering mountain ranges like the Himalayas, which provide habitats for a wide range of species adapted to different elevations. These mountains also act as barriers, limiting the movement of species and influencing patterns of biodiversity.
- Volcanic Activity: Volcanic eruptions can create new landforms such as islands, lava fields, and volcanic cones, which serve as both habitats and barriers. Islands, for example, often harbor unique ecosystems with endemic species adapted to their isolated environments. At the same time, the surrounding ocean can act as a barrier, limiting the dispersal of species between islands and mainland habitats.
- Erosion and Weathering: Processes like erosion by water, wind, and ice, as well as chemical weathering, gradually shape the Earth’s surface, creating a variety of habitats and barriers. River valleys, for instance, provide fertile habitats for plants and animals, while also serving as natural barriers that restrict movement between different regions. Similarly, coastal erosion can form cliffs and beaches, shaping habitats for marine and terrestrial species and creating barriers to coastal development.
- Water Bodies: Rivers, lakes, oceans, and other water bodies play a crucial role in shaping habitats and barriers. Rivers, for example, can create riparian habitats along their banks, supporting diverse plant and animal communities. They can also act as barriers, limiting the movement of species between different watersheds. Similarly, oceans can create barriers to dispersal for marine species, such as deep-sea trenches and currents that influence migration patterns.
- Climate and Geographical Features: Geological features such as latitude, altitude, and proximity to coastlines influence local climates and contribute to the formation of habitats and barriers. For instance, high-altitude habitats like alpine meadows have distinct ecological characteristics compared to lowland forests, creating barriers to species movement and promoting speciation.
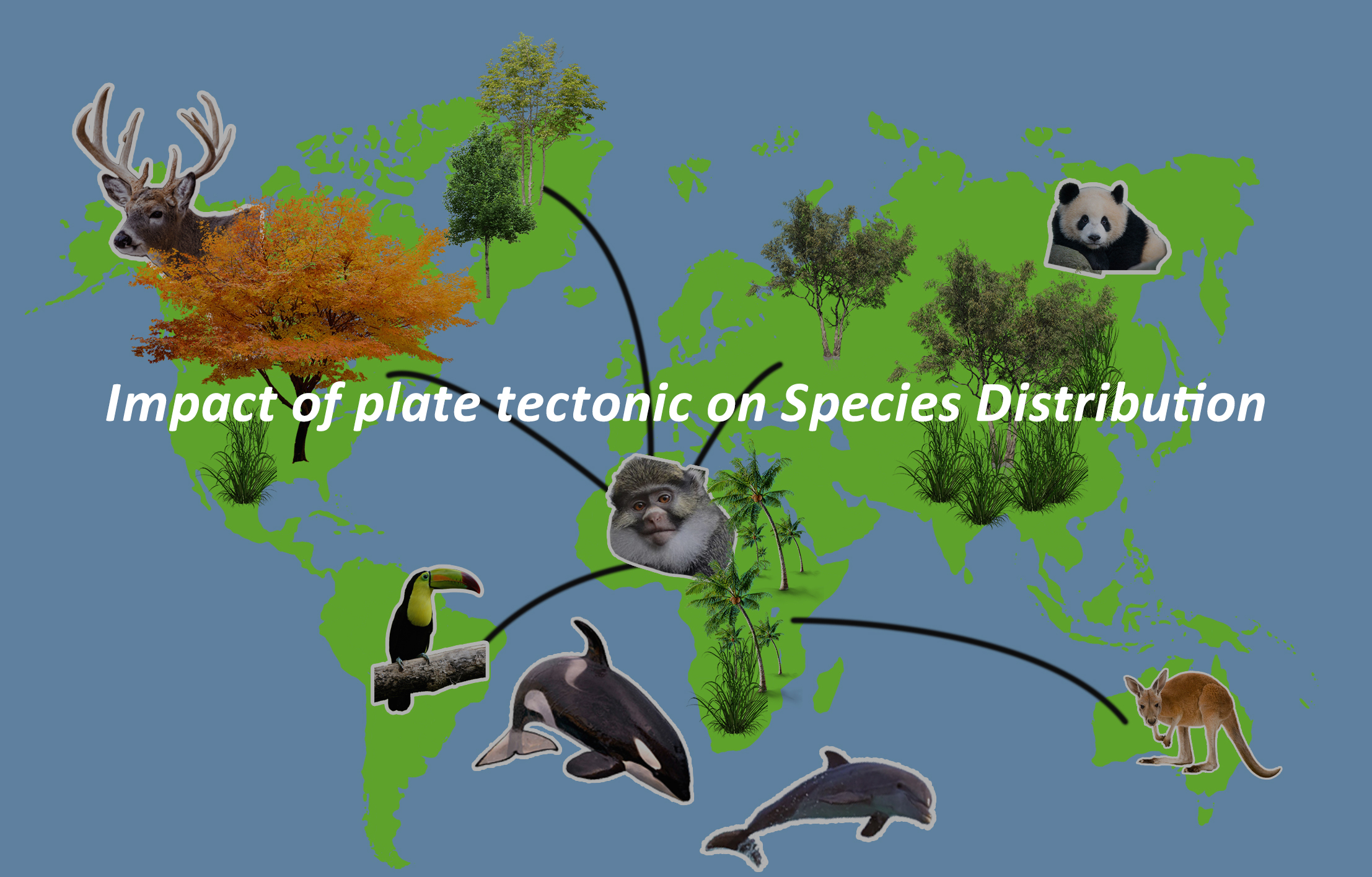
Impact of plate tectonic on Species Distribution
Geological processes have a significant impact on species distribution, including dispersal, isolation, speciation, and adaptive radiation. Here’s how.
A. Dispersal and Isolation: Geological features such as mountain ranges, rivers, and oceans can act as barriers to the movement of species, leading to dispersal limitations and isolation. For example, the Andes Mountains in South America have restricted the movement of many species, leading to the formation of distinct ecosystems on either side of the range.
Also, Islands, formed through volcanic activity or continental drift, often exhibit high levels of endemism due to isolation. Species that colonize islands may undergo adaptive radiation, diversifying into multiple unique forms to exploit available ecological niches. The Galápagos Islands, for instance, are renowned for their diverse array of endemic species resulting from isolation and adaptive radiation.
B. Speciation and Adaptive Radiation: Geological events such as continental drift and the formation of mountain ranges can lead to the isolation of populations, promoting speciation—the process by which new species arise. For example, the uplift of the East African Rift Valley has isolated populations of plants and animals, contributing to the evolution of distinct species adapted to different habitats.
Additionally, Adaptive radiation occurs when a single ancestor species diversifies rapidly to occupy various ecological niches. Geological events that create new habitats or alter existing ones can trigger adaptive radiation. An iconic example is the radiation of Darwin’s finches in the Galápagos Islands, where different species evolved to exploit various food sources and habitats.
Case Studies and Examples of the impact of geological processes on species distribution.
- The Hawaiian Islands: The Hawaiian archipelago provides a compelling case study of the impact of geological processes on species distribution. Each island in the chain represents a unique habitat, fostering adaptive radiation and the evolution of numerous endemic species, such as the honeycreeper birds and silversword plants.
- Madagascar: Madagascar’s separation from mainland Africa millions of years ago has led to its isolation and the evolution of an extraordinary array of endemic species, including lemurs, chameleons, and baobab trees.
- Great Rift Valley: The Great Rift Valley in East Africa has played a crucial role in shaping the distribution and evolution of species, acting as a barrier and driver of speciation. It has contributed to the diversification of iconic African fauna, such as rift valley lakes’ cichlid fish species.
- Antarctic isolation: The isolation of Antarctica due to continental drift and the formation of the Southern Ocean has led to the evolution of unique Antarctic fauna, including penguins, seals, and krill, adapted to extreme cold and isolation.
Biogeographic Realms: Mapping Plate Tectonics’ Influence
Biogeographic Realms refer to large areas of the Earth’s surface defined by distinct ecological and evolutionary characteristics of their flora and fauna. These realms are shaped by a combination of factors, including climate, geography, and geological history, with plate tectonics playing a significant role in their formation and evolution.
A. Wallace’s Realms and Beyond: Alfred Russel Wallace, a 19th-century naturalist, was one of the pioneers in defining biogeographic realms. He identified six major biogeographic realms based on the distribution of animal species. These realms are:
- Nearctic Realm: North America, Greenland, and the northern part of Mexico.
- Palearctic Realm: Europe, Asia (excluding the Indian subcontinent), and North Africa.
- Neotropical Realm: Central and South America.
- Afrotropical Realm: Sub-Saharan Africa.
- Indo-Malayan Realm: Indian subcontinent, Southeast Asia, and Indonesia.
- Australian Realm: Australia, New Guinea, and neighboring islands.
In addition to Wallace’s realms, modern biogeographers have further refined these divisions and identified additional realms, such as the Oceanian Realm, encompassing the Pacific Islands.
B. Notable Features of Different Realms: Each biogeographic realm has its own unique characteristics in terms of climate, vegetation, and animal life. For example:
- The Neotropical Realm is known for its high biodiversity, including the Amazon Rainforest, which is the most extensive tropical rainforest in the world.
- The Afrotropical Realm includes diverse habitats such as savannas, deserts, and tropical forests, supporting iconic species like elephants and lions.
- The Indo-Malayan Realm is renowned for its tropical rainforests, coral reefs, and unique wildlife, including tigers, orangutans, and elephants.
- The Australian Realm is characterized by its distinctive flora and fauna, including marsupials like kangaroos and unique ecosystems such as the Great Barrier Reef.
C. Plate Tectonics’ Role in Defining Realms: Plate tectonics have played a crucial role in shaping the distribution of continents and oceans over geological time scales, which in turn has influenced the evolution and distribution of species. The movement of tectonic plates has led to the formation of barriers (such as mountain ranges and oceans) that have isolated populations, leading to speciation and the development of distinct biotas within different regions.
For example:
- The separation of South America from Africa by the opening of the Atlantic Ocean facilitated the evolution of distinct faunas in these regions, leading to the unique biodiversity found in the Neotropical and Afrotropical realms.
- The collision of the Indian subcontinent with the Eurasian plate resulted in the uplift of the Himalayas, creating barriers that isolated species in the Indo-Malayan realm and led to the development of distinct biotas in South Asia and Southeast Asia.
Plate tectonics provide the underlying framework that has shaped the distribution and diversity of life on Earth, contributing to the formation of biogeographic realms and the patterns of species distribution observed today.
Human Influence and Conservation Implications
(a.) Anthropogenic Impact on Biogeographic Regions: Human activities have a profound impact on biogeographic regions, those areas with unique collections of plants and animals. This impact stems from various anthropogenic factors such as habitat destruction, pollution, introduction of invasive species, and climate change. Habitat destruction, including deforestation and urbanization, directly reduces the available habitats for many species, leading to loss of biodiversity and fragmentation of ecosystems.
Pollution, whether from industrial waste, agricultural runoff, or plastic pollution, contaminates ecosystems, affecting both terrestrial and aquatic life. Additionally, the introduction of invasive species by humans can disrupt native ecosystems, outcompeting native species and altering the balance of biodiversity. Climate change, largely driven by human activities such as burning fossil fuels, contributes to shifts in temperature and precipitation patterns, altering habitats and affecting species distributions.
(b.) Conservation Hotspots and Endemism: Conservation hotspots are areas with exceptionally high levels of biodiversity that are also under significant threat from human activities. These hotspots are crucial for conservation efforts because they harbor a large number of endemic species, which are species found nowhere else in the world. Protecting these areas is vital for preserving Earth’s biodiversity and ensuring the survival of unique species.
Endemism, or the presence of endemic species, is often linked to specific biogeographic regions shaped by plate tectonics. These regions have distinct environmental conditions that have allowed species to evolve in isolation, resulting in unique biodiversity hotspots. Conserving these hotspots and endemic species is essential for maintaining ecological balance and protecting Earth’s natural heritage.
(c.) Long-term Sustainability Strategies:
Long-term sustainability strategies that are essential for preserving biogeographic regions and mitigating the impacts of human activities and environmental changes. These strategies is focused on striking a balance between human needs and the conservation of biodiversity. Key approaches include:
- Habitat Protection and Restoration: Protecting natural habitats and restoring degraded areas help maintain ecosystem health and support diverse plant and animal species.
- Sustainable Land Use Planning: Implementing land use policies that prioritize conservation, minimize habitat fragmentation, and promote sustainable practices such as agroforestry and responsible agriculture.
- Biodiversity Conservation: Implementing measures to protect endangered species, conserve genetic diversity, and establish protected areas to safeguard critical habitats.
- Climate Change Mitigation and Adaptation: Developing strategies to reduce greenhouse gas emissions, promote renewable energy sources, and adapt to the impacts of climate change on biogeographic regions.
- Community Engagement and Education: Involving local communities in conservation efforts, raising awareness about the importance of biodiversity, and promoting sustainable livelihoods that support ecosystem conservation.
- International Cooperation: Collaborating across borders to address transboundary conservation challenges, share knowledge and resources, and implement global agreements such as the Convention on Biological Diversity.
- Research and Monitoring: Conducting scientific research to better understand the dynamics of biogeographic regions, monitor changes over time, and inform evidence-based conservation practices.
By implementing these long-term sustainability strategies, we can work towards maintaining the integrity and resilience of biogeographic regions, ensuring their continued existence for future generations.
Future Prospects and Research Directions
(a.) Technological Advances and Understanding: Technological advances play a crucial role in enhancing our understanding of plate tectonics and its influence on biogeographic regions. Here are some ways technology contributes:
- Remote Sensing: Satellite imagery and remote sensing technologies allow scientists to monitor Earth’s surface changes, such as plate movements, volcanic activity, and land use changes, on a global scale.
- Geographic Information Systems (GIS): GIS tools can help analyze spatial data, such as species distribution, habitat mapping, and land use patterns, to study the relationship between plate tectonics and biogeographic regions.
- Seismic Monitoring: Seismological instruments and networks help detect and study earthquakes, providing valuable data on plate boundaries, crustal movements, and seismic hazards.
- Paleomagnetism: Advances in paleomagnetic techniques enable scientists to study the magnetic properties of rocks, providing evidence for past plate movements and continental drift.
- Geophysical Surveys: Technologies like sonar and seismic reflection profiling help map the seafloor and study oceanic plate movements, leading to insights into marine biogeography and evolution.
- Computer Modeling: High-performance computing allows scientists to simulate plate tectonic processes, climate dynamics, and species distribution models, enhancing our predictive capabilities and understanding of complex Earth systems.
- Data Integration: Integrating multidisciplinary datasets from various sources, such as geological surveys, biological inventories, and climate models, facilitates comprehensive analyses and a holistic understanding of plate tectonics’ role in shaping biogeographic regions.
(b.) Predictions in the Face of Climate Change: In the face of climate change, predictions regarding the impact on biogeographic regions in relation to plate tectonics are crucial. Here’s how they might unfold:
- Shifts in Species Distribution: As temperatures rise and habitats change, species may migrate to higher latitudes or elevations to seek suitable conditions. Plate tectonics can influence the movement of species by creating barriers or corridors for dispersal.
- Altered Habitat Suitability: Climate change may lead to shifts in vegetation zones and the availability of suitable habitats for different species. Plate tectonics can influence the distribution of habitats over geological timescales, affecting species’ abilities to adapt to changing conditions.
- Increased Extinction Risk: Species that are unable to adapt or migrate to new habitats may face increased extinction risk due to climate change. Plate tectonics can influence patterns of species diversity and endemism, which may exacerbate the loss of unique and specialized habitats.
- Rising Sea Levels: Climate change-induced sea-level rise can inundate coastal habitats, impacting marine and terrestrial species. Plate tectonics play a role in shaping coastlines and determining the vulnerability of coastal ecosystems to sea-level rise.
- Extreme Weather Events: Climate change is expected to increase the frequency and intensity of extreme weather events such as hurricanes, droughts, and heatwaves. Plate tectonics can influence the geographical distribution of these events and their impacts on biogeographic regions.
- Ecosystem Services Disruption: Climate change can disrupt ecosystem services provided by biogeographic regions, such as water purification, pollination, and carbon sequestration. Plate tectonics may influence the resilience of ecosystems to climate change-induced disturbances.
(c.) Integrating Plate Tectonics into Conservation Efforts: The art of Integrating plate tectonics into conservation efforts can enhance our understanding of biodiversity patterns, ecosystem dynamics, and conservation priorities. Here’s how this integration can occur:
- Identifying Priority Areas: Plate tectonics influences the distribution of habitats, species, and ecosystems over geological timescales. By considering the geological history and tectonic processes of an area, conservationists can identify priority regions for conservation based on their unique biogeographic characteristics and biodiversity hotspots. One historical example of this is the Galápagos Islands. These islands, located in the Pacific Ocean, were formed by volcanic activity associated with the movement of tectonic plates over millions of years. Charles Darwin’s visit to the Galápagos Islands in 1835 played a pivotal role in shaping his theory of evolution by natural selection. He observed unique species of plants and animals adapted to the diverse habitats found on the islands, leading him to propose that species had evolved over time in response to local environmental conditions. Today, the Galápagos Islands are recognized as a UNESCO World Heritage Site and a biodiversity hotspot. Conservation efforts in the Galápagos prioritize protecting the islands’ unique ecosystems and endemic species, which have evolved in isolation due to their geological history and geographic isolation. By considering the islands’ geological origins and tectonic processes, conservationists can identify priority areas for conservation and management to preserve the islands’ biodiversity and ecological integrity.
- Protecting Evolutionary Processes: Plate tectonics shapes landscapes and drives evolutionary processes such as speciation, extinction, and adaptive radiation. Conservation efforts can focus on preserving areas with high evolutionary potential, such as regions with active tectonic activity or geological features that promote genetic diversity and evolutionary innovation. A good historical example of protecting evolutionary processes influenced by plate tectonics is the Great Rift Valley in East Africa. This vast geological feature, stretching from the Red Sea to Mozambique, was formed by the divergent movement of tectonic plates, creating deep valleys, steep cliffs, and volcanic activity. The Great Rift Valley is renowned for its extraordinary biodiversity and role in the evolutionary history of humans and other organisms. Fossil discoveries in the region, such as those of early hominids like “Lucy” (Australopithecus afarensis), have provided crucial insights into human evolution. Conservation efforts in the Great Rift Valley focus on preserving its unique ecosystems and geological heritage. National parks and protected areas, such as Serengeti National Park in Tanzania and Maasai Mara National Reserve in Kenya, have been established to safeguard the region’s diverse flora and fauna. These conservation initiatives aim to maintain habitat connectivity, prevent habitat fragmentation, and mitigate human activities that threaten the integrity of the Rift Valley’s ecosystems.
- Mitigating Anthropogenic Impacts: Human activities, such as urbanization, deforestation, and pollution, can disrupt natural ecosystems and threaten biodiversity. Integrating knowledge of plate tectonics into conservation planning can help identify areas at risk of habitat degradation or fragmentation due to human activities and prioritize conservation actions to mitigate these impacts. A historical example of integrating knowledge of plate tectonics into conservation planning to mitigate anthropogenic impacts is the case of the Appalachian Mountains in eastern North America. Throughout history, the Appalachian region has been subjected to extensive deforestation, urbanization, and industrial development, leading to habitat loss, fragmentation, and degradation. However, by understanding the underlying geology and tectonic history of the region, conservationists have been able to prioritize conservation efforts and mitigate the impacts of human activities on biodiversity. The Appalachian Mountains formed as a result of the collision of tectonic plates during the assembly of the supercontinent Pangaea hundreds of millions of years ago. The complex geological history of the region has created a diverse range of habitats, including old-growth forests, wetlands, and high-elevation ecosystems, which support a rich array of plant and animal species. Conservation initiatives in the Appalachian region have integrated knowledge of plate tectonics to identify areas at risk of habitat degradation or fragmentation due to human activities such as logging, mining, and urban expansion. By prioritizing conservation actions in key areas with high biodiversity value and ecological significance, such as designated national parks, wildlife refuges, and protected corridors, conservationists have worked to preserve critical habitats and maintain connectivity between fragmented landscapes. For example, the Appalachian Trail, a long-distance hiking trail that spans over 2,000 miles along the Appalachian Mountains, serves not only as a recreational resource but also as a conservation corridor that connects diverse ecosystems and facilitates the movement of wildlife across the region. By integrating knowledge of plate tectonics into conservation planning, stakeholders in the Appalachian region have been able to develop more effective strategies for protecting biodiversity, preserving ecosystem services, and promoting sustainable land use practices in the face of ongoing anthropogenic pressures.
- Facilitating Connectivity: Plate tectonics influences the connectivity of habitats and the movement of species across landscapes. Conservation strategies can incorporate habitat corridors and connectivity networks that account for geological features and tectonic processes to facilitate species dispersal, gene flow, and population resilience in the face of environmental change. One historical example of this is the formation of the Isthmus of Panama around 3 million years ago due to plate tectonics. It connected North and South America, facilitating the exchange of flora and fauna, known as the Great American Interchange. This land bridge allowed species to disperse and colonize new habitats. Today, conservation strategies can emulate this by incorporating habitat corridors that consider geological features. Protecting key areas along such corridors promotes species dispersal, gene flow, and population resilience in the face of environmental change, contributing to biodiversity conservation across landscapes shaped by plate tectonics.
- Promoting Resilience to Climate Change: Plate tectonics can influence the topographic and climatic diversity of biogeographic regions, which in turn affects their resilience to climate change. Conservation efforts can focus on protecting areas with diverse microclimates, elevation gradients, and geological formations that provide refugia for species vulnerable to climate change-induced stressors.
- Fostering Transboundary Collaboration: Plate tectonics often transcend political boundaries, making conservation efforts in biogeographic regions inherently interconnected. Integrating plate tectonics into conservation planning encourages collaboration among stakeholders across borders to address shared conservation challenges, promote habitat connectivity, and enhance ecosystem resilience at regional scales. An exemplary instance of transboundary collaboration in conservation related to plate tectonics is seen in the preservation of the Virunga Massif, straddling the Democratic Republic of the Congo (DRC), Rwanda, and Uganda. Despite political boundaries and regional conflicts, concerted efforts by conservation organizations, governments, and local communities have aimed to safeguard the region’s biodiversity. Initiatives like the Greater Virunga Transboundary Collaboration (GVTC) have facilitated joint patrols, habitat conservation, and sustainable tourism development across borders. By acknowledging the geological and ecological interconnectivity of the Virunga Massif, conservation strategies have effectively addressed shared challenges, such as habitat loss and poaching, while promoting habitat connectivity and ecosystem resilience. This collaborative approach underscores the importance of integrating plate tectonics into conservation planning to foster cooperation and enhance the sustainable management of biogeographic regions.
In conclusion, the dynamic interplay between plate tectonics and biogeographic regions highlights the complex relationship between Earth’s geological processes and biodiversity. Plate tectonics has a major impact on the natural world, shaping landforms, ecosystems, and species distribution. By integrating plate tectonics into conservation efforts, we can better understand the interconnectedness of ecosystems across borders and develop comprehensive strategies to preserve biodiversity and promote ecosystem resilience.
From fostering transboundary collaboration to mitigating the impacts of climate change, incorporating knowledge of plate tectonics enriches our conservation approach and enhances our ability to protect the planet’s natural heritage for future generations.
Also, continued research, collaboration, and innovation will be essential in addressing the complex conservation challenges posed by ongoing environmental changes and human activities. Through collective action and stewardship, we can strive to safeguard the integrity and diversity of biogeographic regions, ensuring a sustainable future for all life on Earth.
Here are recommended reading resources and publications for further studies: